Research
Scientific Orientation
The focus of our research group lies on the investigation of the photophysical and -chemical properties of transition metal complexes, in particular in their d10 electron configuration, for potential applications in OLEDs, as photocatalysts, sensors or in the area of quantum communication. Our main motivation is the conceptual development of design motifs to achieve the desired properties of the transition metal compounds for a given application, where we also strive to expand the general understanding of excited states in the literature. This highly interdisciplinary orientation of the research of the Steffen group at the interface between classical organometallic chemistry, photophysics/-chemistry, materials science, (photo)catalysis and theoretical chemistry, led to the establishment of many national and international collaborations and an excellent local instrument infrastructure (see Equipment and Infrastructure), providing students and future independent scientists with the opportunity to gain a very broad education with various emphases. In the following, some general aspects of our work and specific projects will be highlighted.
Importance of photoactive transition metal complexes
Light-absorbing and -emitting transition metal complexes are of utmost importance for the development of innovative photonic applications because in stark contrast to common organic molecules, that fluoresce on the nanosecond timescale, strong spin-orbit coupling (SOC) can be mediated by the metal atom leading to (ultra-)fast intersystem crossing (ISC) Sn→Tn. The thus accessible triplet state T1 exhibits fundamentally different properties than the singlet excited state S1. For instance, in dependence of the specific operative SOC, which reduces the spin-forbidden character of the phosphorescence T1→S0 in metal complexes, high quantum yields with luminescence lifetimes of a few microseconds to several milliseconds can be achieved. Such long lifetimes of the excited states are beneficial for sensor applications, e.g. detection of molecular oxygen or small molecules, and can be used for time-gated biological imaging. Furthermore, transition metal complexes are both much stronger reducing and oxidizing agents in their excited triplet state than in the ground state S0, which, together with the long lifetime of the T1 state, provides the possibility to employ them in photo-redox-catalysis for single electron transfer (SET). Due to the generally lower energy of the T1 state in comparison to the S1 state, emission via phosphorescence also allows to access near-infrared (NIR) emitters. Phosphorescent transition metal compounds are being considered as very promising candidates for emitter materials in light emitting diodes (LEDs), as the electron-hole recombination process forms 75% triplet states, which would decay non-radiatively if only organic fluorophores would be used so that a maximum internal device quantum efficiency of only 25% could be achieved. Metal complexes can harvest triplet excitons with high radiative rate constants, so that highly efficient LEDs working at the theoretical efficiency maximum become possible.
d10-Metal complexes of the abundant elements as attractive alternatives
As ISC as well as the radiative rate constants depend, among other factors, on the operative SOC and as its constant is proportional to Zeff4/n3 (with Zeff being the effective nuclear charge and n being the principal quantum number of the valence electron) – i.e. heavy atom effect – research regarding application of photoactive transition metal compounds has been concentrated in particular on heavy but rare 4d and 5d elements such as RuII, OsII, IrIII and PtII. However, in these d6 and d8 complexes, low-energy metal-centered (MC) d-d* states can lead to very fast, non-radiative decay and present a serious challenge to stabilizing the triplet states.
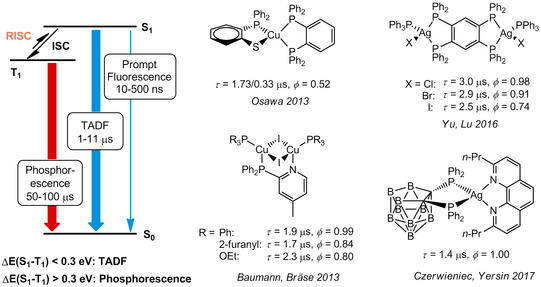
The problem of the undesirable rapid and radiation-free deactivation of the triplet states, as well as the cost aspects of rare elements, can be solved by the elegant approach of using d10 metal complexes of common elements in which no MC(d-d*) transitions occur, such as copper or zinc. The significantly lower SOC of these light elements compared to 5d metals "traditionally" used for luminescent complexes, which usually leads to high radiation constants of the T1 state, can be compensated by so-called thermally activated delayed fluorescence (TADF). This means the thermal repopulation of the S1 state at room temperature followed by fluorescence S1→S0, which bypasses the formal spin-forbidden triplet emission T1→S0 and enables high radiation constants (or short emission lifetimes) with high quantum yields. This mechanism is particularly common for Cu(I) compounds (Fig. 1).
A further advantage of d10 transition metal complexes over d6 octahedral or square-planar d8 compounds is their flexible coordination geometry (linear, trigonal, tetrahedral), which opens up a wider range of possibilities to influence the excited states in their nature and their photophysical and photochemical properties. This includes their ability to undergo chemical transformations, electron or energy transfer to suitable substrates and thus to act as photo(redox) catalysts.
Challenges and own motivation/goals
The choice of π chromophore ligands for d10 metal compounds is very limited. For example, most photoactive copper(I) and zinc(II) compounds are based primarily on phosphine, diimine and halide ligands, while very little is known about luminescent d10 metal NHC complexes (NHC = N-heterocyclic carbene). In addition, few general structure-property relationships have been developed with respect to TADF, which is still difficult to predict a priori, especially since d10 metals can assume different coordination geometries (linear, trigonal, tetrahedral), which may have an impact on operative SOC and TADF. The same is true for the targeted design of chiroptic properties, which should be expressed in particular in circularly polarized triplet luminescence with high radiation constants, as well as the redox potentials and energy transfer capability of the excited states. For this reason, the basic understanding of the photophysically excited state behaviour of d10 metal systems is still underdeveloped and the potential for possible applications has not yet been clarified.
Our group aims to contribute to close these intellectual gaps by developing new design motifs and metal complex classes for photonic applications. Against this background, the basic motivation and goals of our work can be summarized as follows:
- Development of structure-property relationships of the photophysical properties of d10 transition metal complexes, by
a) Establishment of new π chromophore ligands for d10 metal compounds
b) Development of the photophysics of d10 metal-carbene complexes
c) Investigation of metallophilic interactions in excited states - Transfer of the findings to material science applications
- Development of new photocatalytic systems
Examples of current projects
I) Highly efficient (chiroptical) (NIR-)emitter
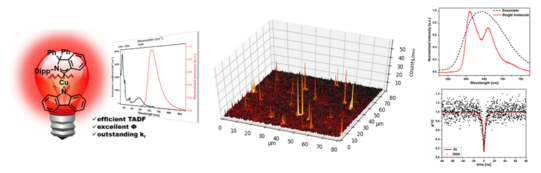
The development of near-infrared emitters is of great importance for the tele- and quantum communication sector. Phosphorescent triplet states of metal complexes are particularly suitable in the device area because their energy is generally lower than that of excited fluorescent singlet states. In the NIR, however, non-radiative deactivation is usually faster than luminescence. Therefore, we investigate rigid (chiral) ligands with low energy LUMOs for effective MLCT with high spin-orbit coupling or very efficient TADF. We test suitable candidate compounds with high radiation constants and moderate to good quantum yields in plasmon resonance structures as non-classical single photon sources.
J. Am. Chem. Soc. 2020, 142, 8897
Angew. Chem. Int. Ed. 2015, 54, 1570
Inorg. Chem. 2017, 56, 8996
Chem. Eur. J. 2017, 23, 2206
II) Cooperative effects in multi-metal complexes
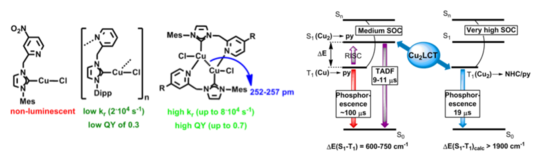
Metallophilic interactions allow the participation of several metal centers in the excited state, increasing the operative spin-orbit coupling for efficient triplet emission, thus accelerating triplet emission via phosphorescence or the TADF process. In addition, multiple, not fully equilibrated excited states can lead to dual emission and allow the design of white light emitters. In this project we investigate the structural parameters of multimetal complexes in the ground state and their influence on luminescence.
Chem. Commun. 2016, 52, 2932
Chem. Commun. 2015, 52, 5019
Angew. Chem. Int. Ed. 2016, 55, 10507
Chem. Eur. J. 2017, 23, 11684
III) Stimulus-responsive phosphorescent materials

Luminescent materials that react to an external stimulus such as mechanical stress by changing photophysical properties (light color, efficiency, lifetime, polarization) can be used as sensors, data storage and molecular switches. While fluorescence emitters are well established in this respect, there are no suitable design criteria for phosphorescent responsive systems so far. We were able to establish a simple new mechanochromic phosphorescence mechanism in the literature, based on the reversible contact pairing of a cationic metal complex with its counterion. Currently, we are investigating different low-coordinated metal complexes and the influence of the anions as a function of their shape and size on the change of properties.
Angew. Chem. Int. Ed. 2018, 57, 13671
IV) Bimetallic photocatalysts for hydroelementation reactions
Photons as energy supply can influence the selectivity of chemical transformations. Metal complexes are used in this field as "antennas", which absorb light and, in the excited state, thanks to their altered redox potential, can transfer electrons to organic substrates (reduction) or oxidize them. In this type of photo redox catalysis, the metal centre is not directly involved in the chemical reaction, but only functions as an electron shuttle. The special advantages of metal-organic catalysis mechanisms, such as bond activation, proximity of substrates, chiral induction, etc., are lost here. We investigate metal-mediated catalytic reactions in which the reaction mechanism is advantageously influenced by light excitation.